Here are a list of the research projects.
Click on project to see more.
Active Research:
(Codename: E.I.T.)
Diffraction limits how tightly light can be focused. This limits resolution in optical microscopy and lithography and sets a minimum volume that can be addressed with a laser beam. Beating the diffraction limit could allow for smaller microchips and for more powerful microscopes. Being able to address a smaller volume could be very useful in quantum computing. We seek to work around the diffraction limit by using the nonlinear power dependence of Electromagnetically Induced Transparency (EIT) to transfer atoms in very small regions, smaller than the diffraction limit. EIT can be explained as a non-linearity generated by the quantum interference between an atom's energy levels. Our most recent experimental work has localized atoms to regions ~100nm wide, 1/8 the wavelength of our lasers! In addition, in our most numerical work, we construct a high-resolution phase gate structure with EIT and we report theoratical resolution at ~4nms on single atom adressing.
Current Students: Utku Saglam
Past Students:
Dipto Das,
Jared Miles,
Zach Simmons,
Nick Proite
Selected Related Article:
Axions are low mass hypothetical particles that are postulated to exist outside the Standard Model. Their existence would solve multiple puzzles in particle physics, such as the strong CP(charge conjugation symmetry and parity symmetry) problem. Because of their weak interactions, they are hard to detect and none have been detected as of yet. We are considering an experimental scheme to create and detect and detect axions using laser-based four-wave mixing, which is mediated by the hypothetical axion field. In our current scheme, two intense laser beams are confined toa waveguide and drive the axion generation. In the waveguide structures, we predict guided axion waves which we call "axitons". These axitons are solutions to the Klein-Gordon field equation that are guided by the laser beams. These axiton waves later on can couple to a nearby detection fiber, consequently mixing with another laser for detection.
Current Students: Utku Saglam, Shay Inbar
Selected Related Articles:
Generation and detection of axions using guided structures
(Theory - 2022)
(Codename: Project Rainbow)
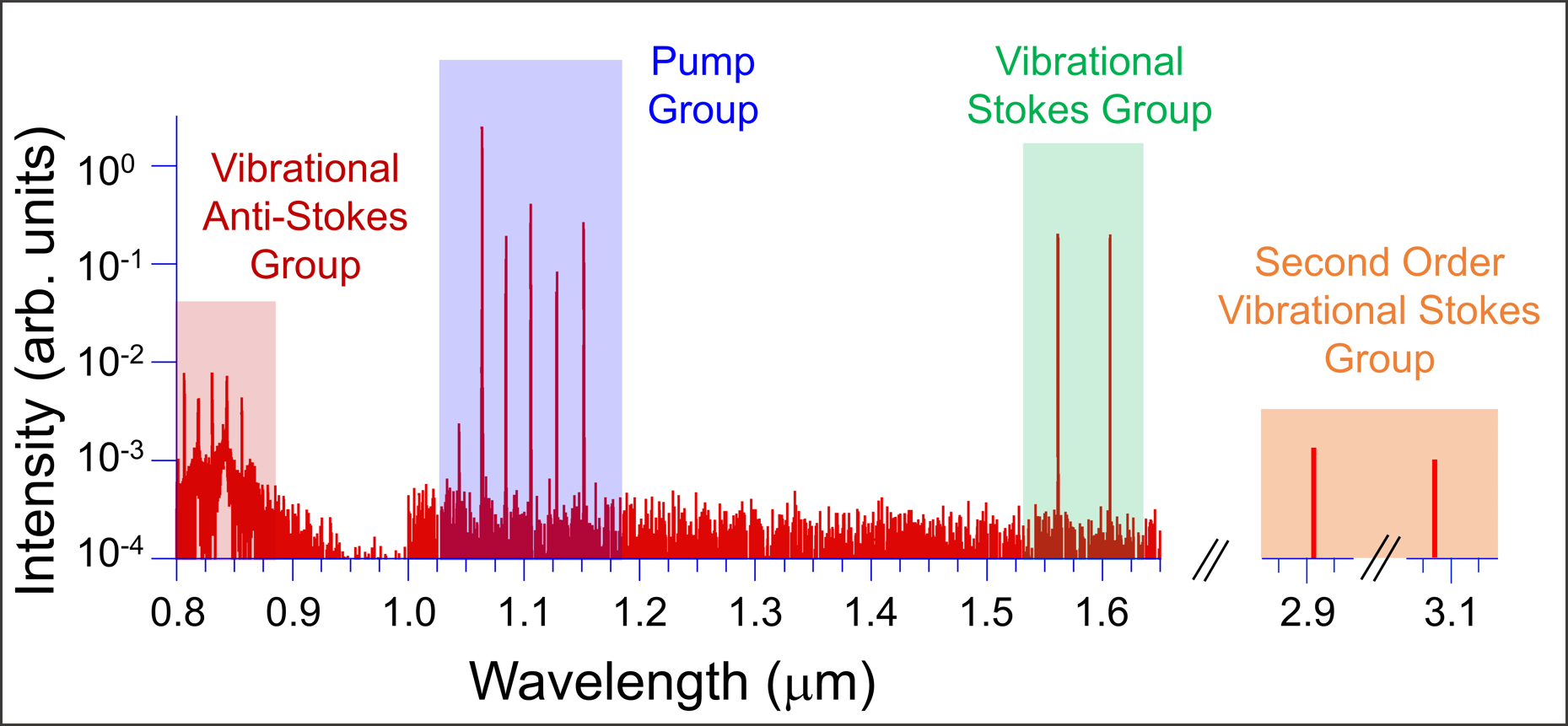
Our ultrafast project focuses on the modulation of light at molecular frequencies. Our modulator takes incident laser light and shifts the frequency of a fraction of it, turning one monochromatic laser beam into multiple coherent beams of different colors. By stimulating Raman scattering on vibrational and rotational molecular transitions, we modulate continuous-wave light at frequencies up to 100 THz. This shifts the wavelength by up to hundreds of nanometers. Using this technique, we are working toward generating an extremely broad coherent light source. This "white laser" will have several million Fourier components spanning the optical spectrum from the far ultraviolet to the infrared. In addition to a variety of spectroscopic applications, such a broad coherent source would permit the exploration of ultrafast processes. Exciting potential uses include the generation of light pulses that last less than a femtosecond and the creation of arbitrary waveforms at optical frequencies.
We have previously demonstrated modulation of light in high-finesse optical cavities filled with a molecular gas. We and are now in the process of extending this technique to solid-state microresonators that support whispering gallery modes.
Current Students: None (Interested? Contact us!)
Past Students: David Gold,
Josh Karpel,
Josh Weber,
Tyler Green
Selected Related Articles:
High-power near-CW Raman lasing in mm-sized glass disks
(Experiment - 2022)
Prospects for Continuous-Wave Molecular Modulation in Raman-Active Microresonators (Theory - 2019)
Phase-dependent ionization of hydrogen
by intense sub-cycle pulses (Theory - 2018)
Continuous-wave modulation of a femtosecond
oscillator using coherent molecules (Experiment - 2018)
Broadband
spectrum generation using continuous-wave Raman
scattering (Experiment - 2013)
Continuous-Wave Light Modulation at Molecular Frequencies
(Experiment - 2010)
High-frequency modulation of continuous-wave laser beams by maximally
coherent molecules (Theory - 2007)
(Codename: Shepherd)
No quantum computer can be completely isolated from the rest of the universe, and so all quantum computers will be subject to decoherence effects from interactions with its environment, causing errors in the computation. Much progress has been made to demonstrate that the errors caused by a wide variety of sources can be corrected for by using extra "qubits" (the quantum analog of a bit) and extra steps in the computation. However, we suspect that a phenomenon known as superradiance is a source of error which cannot be corrected for.
Any system with two energy levels, such as a qubit, has a probability of spontaneously jumping from the higher energy level to the lower one, in a process called spontaneous emission. This kind of error can be corrected for, so long as the rate at which this process occurs is low enough. However, when many two level systems are near each other, the rate of spontaneous emission increases with the number of qubits through the process of superradiance. This means that when the quantum computer is large enough, the rate of spontaneous emission may become so high that it causes errors which cannot be corrected, which would put a limit on the size of a functional quantum computers.
We are currently investigating the phenomenon of superradiance theoretically, computationally, and experimentally to gain a more fundamental understanding of the process and ultimately what implications it may have for quantum computing.
Current Students: Utku Saglam
Past Students: Ben Lemberger,
Dipto Das
Selected Related Articles:
Spatial Coherence of Light in Collective Spontaneous Emission
(Experiment - 2022)
Effect of correlated decay on fault-tolerant quantum computation
(Theory - 2017)
Large Sample Superradiance and Fault-Tolerant Quantum
Computation (Theory - 2015)
Superradiance as a source of collective decoherence in quantum
computers (Theory - 2014)
Dormant Research:
Left-Handed Electromagnetic Waves ▼
(Codename: Project Green Lantern)
Two main challenges to creating a negative index
of refraction at optical frequencies are the need for a high
density of oscillators and the need for a strong magnetic
response at optical frequencies. Using a rare-earth
doped crystal can address both of these issues.
Europium has a strong magnetic dipole transition at 527 nm,
and being in a solid means it has a potential to have a much
higher density than a MOT or a FORT. Our ultimate goal
is to create left-handed waves in a europium doped yttrium
orthosilicate crystal.
Current Students: [your name here!]
Past Students: Zach
Buckholtz, Nick
Brewer, Zach Simmons
Selected Related Articles:
Negative refraction in terbium at ultraviolet frequencies (Theory - 2020)
Linear and Nonlinear Crystal Optics Using the Magnetic Field of Light (Experiment - 2020)
Coherent Magnetic Response at Optical Frequencies Using Atomic Transitions (Experiment - 2017)
Left-handed Electromagnetic Waves in Materials with Induced Polarization and Magnetization (Theory - 2014)
Refrative
Index Engineering▼
There has been much progress in
the last few decades manipulating the optical response of
atomic media to create interesting effects like slow and
stopped light. We aim to engineer the index of refraction
in atomic systems using Raman and related effects. Many
interesting questions arise: Is it possible to create a
large index in an atomic gas while eliminating absorption?
Can we engineer a negative index of refraction in an
atomic system? Applications range from nano-scale optical
microscopy to quantum information to more far-out
applications like creating 'perfect' lenses.
Current Students: [Your name here!]
Past Students: Shay Inbar,
Jared Miles,
Zach Simmons,
Dan Sikes, Nick Proite
Selected Related Articles: